Java Healthcare Projects in Java: Comprehensive Review
Reviewing The Java Healthcare Solutions
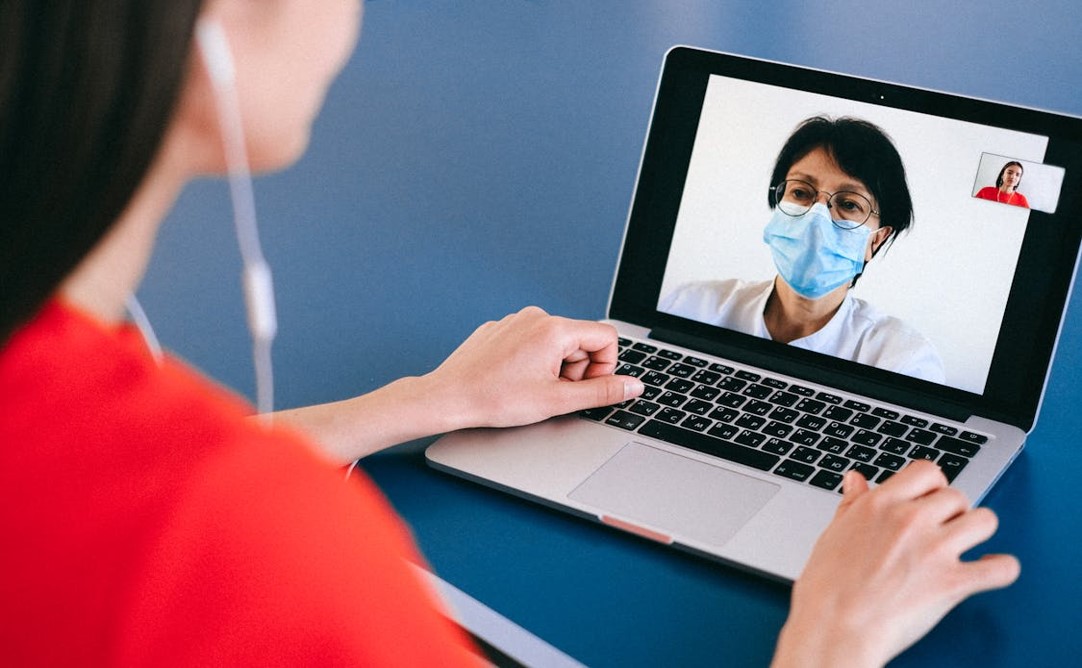
If you’ve ever had anything to do with healthcare tech, you’ve probably heard about Java healthcare solutions. Java is a powerhouse in the tech world, and it’s now making waves (and huge ones!) in healthcare, too. Let’s find out why it’s a top pick for healthcare projects.
Advantages of Using Java for Healthcare Projects?
So, why hire Java developers? Because the framework has A LOT to offer. You’ll see it yourself now.
Security
First off, security. Healthcare data is sensitive. You know too well how personal health information (PHI) is a prime target for cyberattacks today. The framework, in turn, offers cool security features like
- advanced authentication,
- encryption,
- and secure APIs.
So it’s a really strong contender for any e-healthcare management system Java project.
Scalability
Healthcare systems handle lots of data. There are patient records, there are medical images, and whatever else. Scalability is, in fact, a guarantee that your wireless healthcare system project Java can grow when you need it to grow. And it doesn’t matter if you’re a small clinic or a large hospital network. The framework’s got the resources to handle any (okay, almost any) data scope.
Cross-Platform Compatibility
Another big plus is compatibility. That is, your e-healthcare project in Java runs on various devices and operating systems. Why is that so vital? Well, that’s because systems in healthcare settings need to communicate across different platforms. And thanks to Java, doctors can access patient records on tablets, desktops, or even smartphones.
Community and Support
Lastly, Java has a massive developer community and a wealth of libraries and frameworks. This means if you’re working on a healthcare management system Java project, you’ve got plenty of resources and support to lean on. Need a specific functionality? 90% that there’s a library for that.
Java Software Engineering in Medical Research
-
Data Management and Analytics
Java has great data handling capabilities, thanks to which, it can manage and analyze massive datasets. Think about clinical trials or patient studies. With Java, researchers can collect, store, and analyze data. Based on this, they further draw insights that drive medical advancements.
For instance, in a Java healthcare project, you might use Java-based tools to sift through patient records and identify persistent trends. This knowledge can then inform treatment protocols or highlight the efficacy of new medications. Because the framework can integrate with big data technologies like Hadoop and Spark, its data processing power is, indeed, huge.
-
Medical Imaging Tools
Tools for MRI, CT scans, and X-rays require robust software for image processing and analysis. Java, in turn, has an object-oriented programming nature so it’s fit for developing sophisticated imaging tools that provide clear, precise images.
Imagine working on an e-healthcare management system project in Java, where you need to integrate imaging functionalities. The framework’s extensive libraries and APIs allow you to develop applications that handle image storage, enhancement, and analysis. This improves diagnostic accuracy and speeds up the process. It’s thus beneficial for both doctors and patients.
-
Remote Healthcare
The shift towards remote healthcare has been significant, especially post-pandemic. And Java can help with developing telemedicine platforms, too. These platforms will be reliable, secure, and capable of real-time data transmission. They will be 100% fit for remote consultations, patient monitoring, and follow-ups.
Consider an e-healthcare project in Java where patients can
- interact with doctors via video calls,
- share health data through connected devices,
- and receive remote monitoring.
Thanks to the framework’s strong networking capabilities, all this can, indeed, happen (and happen without a hitch).
-
Safety and Confidentiality
Besides all else, the framework can help to develop applications that comply with regulations like HIPAA in the US. This protects patient information and ensures that healthcare providers meet legal standards.
In a healthcare management system Java project, for instance, you can use Java’s security features to implement encrypted data storage, secure data transmission, and robust user authentication mechanisms. This likewise helps to build trust with patients.
Conclusion
As you now see. Java can do quite a lot for the healthcare industry today. First of all, it offers robust security and scalability opportunities. Second, it can play an important role in data management, medical imaging, and remote healthcare. Put simply, its advantages are clear, you just need to use it right.
FAQs
1. How does Java ensure the security of healthcare applications?
It offers features such as advanced authentication, encryption, and secure APIs. These protect sensitive healthcare data in any system.
2. Can Java handle large-scale healthcare data?
Yes, it’s pretty scalable so it can manage vast amounts of data. It’s fit for projects of practically all sizes.
3. Is Java suitable for developing cross-platform healthcare applications?
Absolutely. One of its beneficial traits is cross-platform compatibility. That is, applications run equally well on various devices and operating systems.
4. How does Java contribute to medical imaging solutions?
Its object-oriented programming and libraries support the development of medical imaging tools. The latter, in turn, enhances diagnostic accuracy and efficiency in Java healthcare projects.
5. What role does Java play in remote healthcare?
It shows robust performance and has strong security features. Thanks to these, it enables the development of reliable telemedicine platforms. Such platforms facilitate smooth remote consultations and patient monitoring.